Scientific Objectives
Nitric oxide is an important minor constituent of the upper atmosphere that exhibits strong solar-terrestrial coupling. Nitric oxide directly affects the composition of the ionosphere, the thermal structure of the thermosphere, and may be transported downward into the mesosphere and stratosphere where it can react with ozone. However, significant unanswered questions about nitric oxide remain. The scientific objectives of the Student Nitric Oxide Explorer are:
- To determine how variations in the solar soft X-radiation produce changes in the density of nitric oxide in the lower thermosphere,
- To determine how auroral activity produces increased nitric oxide in the polar regions.
Nitric oxide (NO) has a maximum density of about 3E7/cc near 110 km (see figure below). In the polar region the mean density is several times greater and highly variable sometimes being as much as 10 times larger (see figure below). The importance of nitric oxide in the upper atmosphere is the result of its chemical, electrical, and radiative properties. Nitric oxide is more easily dissociated and ionized than the principal molecular constituents, nitrogen and oxygen. Nitric oxide radiates in the infrared while the major constituents of the atmosphere do not.
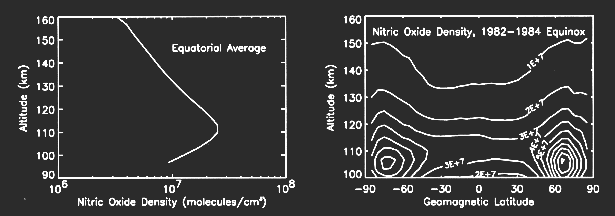
The principal source of nitric oxide in the lower thermosphere is the reaction of energetic nitrogen atoms with molecular oxygen. These nitrogen atoms need to have excess energy, either electronic or kinetic, in order for the reaction with molecular oxygen to proceed rapidly. Nitrogen atoms at normal temperatures in the lower thermosphere produce only a small amount of nitric oxide. Sources of the energetic nitrogen atoms are ionospheric reactions and energetic electron impact on molecular nitrogen. Energetic electrons created by photoionization (photoelectrons) and by auroral particle bombardment (auroral secondary electrons) thus create nitric oxide both by dissociating molecular nitrogen and by ionizing all neutral species, which drives ion-neutral and dissociative recombination reactions that create excited nitrogen atoms.
The hypothesis has been proposed that the variation in the density of low latitude nitric oxide at 110 km is caused by the variation in the solar output of soft X-rays in the wavelength range 2-10 nm [Siskind, et al., 1990]. The reasoning behind this hypothesis is that the absorption coefficients of the atmospheric constituents are such that the solar soft X-rays are absorbed in the 100-120 km altitude region and that the ionization by the soft X- rays produces a copious source of photoelectrons. The hypothesis further states that the solar soft X-rays vary with a greater amplitude than does the solar extreme ultraviolet radiation. The evidence for this hypothesis comes from three years of observations of thermospheric nitric oxide from the Solar Mesosphere Explorer [e.g., Barth, et al., 1988]. The SME observations show that the nitric oxide density at low latitudes varies with the 27-day solar rotation period and with the 11-year solar cycle. The variation of nitric oxide correlates with two solar indices, the solar Lyman alpha irradiance which was measured from the SME spacecraft and the solar 10.7 cm radio flux which is a solar index that is measured from the ground (see figure below).
The first objective of the Student Nitric Oxide Explorer is to test this hypothesis. The solar soft X-ray irradiance in the wavelength range 2-10 nm and the solar extreme ultraviolet radiation between 10 and 31 nm will be measured with a solar X-ray photometer. The solar soft X-rays are expected to vary with a much larger amplitude than the 30.4 nm chromospheric line which is the most important extreme ultraviolet feature for ionization and photoelectron production. The nitric oxide density in the lower thermosphere will be measured with an ultraviolet spectrometer (215-237 nm) that observes the fluorescence of nitric oxide in the gamma bands. The comparison of the measured nitric oxide at low latitudes (25° S – 25° N) between 100 and 120 km with the solar soft X-rays will show whether or not this hypothesis is correct. Further, the comparison of the observations will show the functional relationship between the magnitude of the nitric oxide density and the soft X-ray irradiance (2-10 nm) and the extreme ultraviolet radiation (10-31 nm).
Global observations of nitric oxide from satellites have shown that the maximum amount of nitric oxide occurs in the polar regions centered at geomagnetic latitudes of 65° N and 65° S and in the altitude region between 100 and 110 km. The most plausible explanation is that the polar region nitric oxide is produced by the impact of auroral electrons. When auroral primary electrons (energies 1-10 keV) bombard the atmosphere, they produce large numbers of secondary electrons. Those secondary electrons with energies greater than 13.3 eV are able to dissociate molecular nitrogen and produce energetic nitrogen atoms which in turn produce nitric oxide. Secondary electrons with energy greater than 15.5 eV ionize molecular nitrogen leading to the production of energetic nitrogen atoms. Photochemical theory indicates that any nitric oxide produced in the auroral zone will have a lifetime of greater than a day; thus, it is the auroral activity of the previous day or longer that should determine the amount of nitric oxide present. During the auroral bombardment process, the secondary electrons also excite molecular nitrogen and atomic oxygen to produce auroral emissions in the ultraviolet and visible portions of the spectrum. The intensity of these auroral emissions may be used to determine the flux of the auroral electrons. The theory of auroral excitation is well-developed [e.g., Solomon, 1993]. SME observations of nitric oxide in the polar regions show that there are large variations in nitric oxide density and that these variations are related to auroral activity [Barth, 1990; Barth, 1992]. The above figure shows average nitric oxide density profiles for conditions of low, medium, and high auroral activity. The geomagnetic index Ap was used to sort the observations into low, medium, and high activity; however, there is not a satisfactory quantitative relationship between nitric oxide density and the Ap index.
The second objective of SNOE is to determine how auroral activity produces increased nitric oxide in the auroral regions. This will be accomplished by measuring the nitric oxide density with the ultraviolet spectrometer and by measuring the intensity of the ultraviolet aurora with the auroral photometer. The auroral photometer will determine the intensity of the Lyman-Birge- Hopfield (LBH) bands of molecular nitrogen and the atomic oxygen resonance (130.4 nm) and forbidden (135.6 nm) lines, which are excited by auroral electron impact. Since the ultraviolet aurora will be measured on the nightside every orbit (96 min.) in both the north and south hemispheres, a global time history of auroral activity will be determined.