Science
Introduction
Why Study the Sun and How does SORCE Help?
Solar radiation is the dominant, direct energy input into the terrestrial ecosystem; and it affects all physical, chemical, and biological processes. The Sun provides a natural influence on the Earth’s atmosphere and climate. In order to understand mankind’s roles in climate change, the Sun’s impact must first be understood.
SORCE measures the Sun’s output with the use of state-of-the-art radiometers, spectrometers, photodiodes, detectors, and bolometers engineered into instruments mounted on a satellite observatory. The SORCE satellite orbits around the Earth accumulating solar data. Spectral measurements identify the irradiance of the Sun by characterizing the Sun’s energy and emissions in the form of color that can then be translated into quantities and elements of matter. Data obtained by the SORCE experiment will be used to model the Sun’s output and to explain and predict the effect of the Sun’s radiation on the Earth’s atmosphere and climate.
Detailed Science Introduction
Energy from the Sun makes life on Earth possible. Solar energy also drives the Earth’s climate, and slight variations in solar radiance could offset (or increase) global warming. The 23.5° angle between Earth’s spin axis and its orbit about the Sun gives rise to the seasonal cycle, causing the length of the day and the sunlight angle to vary during the year. As a result, summer is much warmer than winter, and the polar regions have continuous daylight during their summer and continuous nighttime during their winter. Also, Earth’s orbit about the Sun is an ellipse, and not a precise circle, with the Earth slightly closer in early January than it is in July. This results in about 7% more sunlight reaching the Earth in January than in July. Both of these seasonal effects, the tilt of the Earth’s axis, and the orbital distance to the Sun, are stable and very predictable; and the resulting annual cycle in sunlight remains the same year after year.
The total solar irradiance, or TSI, along with Earth’s global average albedo, determines Earth’s global average equilibrium temperature. Because of selective absorption and scattering processes in the Earth’s atmosphere, different regions of the solar spectrum affect Earth’s climate in distinct ways. Approximately 20 – 25 % of the TSI is absorbed by atmospheric water vapor, clouds, and ozone, by processes that are strongly wavelength dependent. Ultraviolet radiation at wavelengths below 300 nm is completely absorbed by the Earth’s atmosphere and contributes the dominant energy source in the stratosphere and thermosphere, establishing the upper atmosphere’s temperature, structure, composition, and dynamics. Even small variations in the Sun’s radiation at these short wavelengths will lead to corresponding changes in atmospheric chemistry. Radiation at the longer visible and infrared wavelengths penetrates into the lower atmosphere, where the portion not reflected is partitioned between the troposphere and the Earth’s surface, and becomes a dominant term in the global energy balance and an essential determinant of atmospheric stability and convection. Thus it is important to accurately monitor both the TSI and its spectral dependence.
Variations of the solar radiation field are still largely unknown, but in the visible they are likely far less than one percent. The observations therefore require precision and accuracy that can only be achieved from space. Although the ultraviolet radiation from the Sun varies by much larger factors, its measurement also requires access to space since the radiation does not penetrate the atmosphere. Precise space measurements obtained during the past 20 years imply that TSI varies on the order of 0.1% over the solar cycle (see Figure 1), but with greater variations on a short-term basis. For example, the passage of sunspots over the disk produces 2-4 times that amount. The variation apparently occurs over most time scales, from day-to-day variations up to and including variations over the 11-year solar cycle. How TSI variations are distributed in wavelength is still poorly understood. The largest relative solar variations are factors of two or more at ultraviolet and shorter wavelengths, but the greater total energy available at visible and longer wavelengths makes their small variations of potential importance.
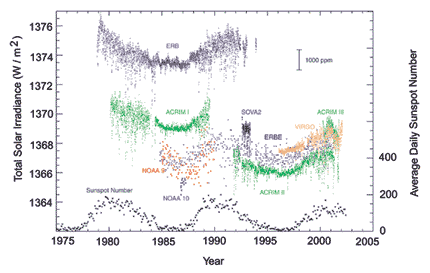
Figure 1: Total Solar Irradiance Coverage
Assuming climate models include a realistic sensitivity to solar forcing, the record of solar variations implies a global surface temperature change on the order of only 0.2° C. However, global energy balance considerations may not provide the entire story. Some recent studies suggest that the cloudy lower atmosphere absorbs more visible and near infrared radiation than previously thought (25% rather than 20%), which impacts convection, clouds, and latent heating. Also, the solar ultraviolet, which varies far more than the TSI, influences stratospheric chemistry and dynamics, which in turn controls the small fraction of ultraviolet radiation that leaks through to the surface.
Figure 2 shows the solar irradiance between 1 nm and 2000 nm where the spectrum is displayed with an effective spectral resolution of 1 nm. This spectrum has the general characteristic of a continuum spectrum throughout, with many absorption features, both lines and absorption edges, and at the shorter wavelengths superposed with emission features. In consideration of the Sun as the source of this emission, the longest wavelengths originate low in the photosphere, and progressing toward shorter wavelengths the emission originates higher and higher in the solar atmosphere, until at the very shortest wavelengths the emission is dominantly from the chromosphere with a few higher temperature lines originating in the solar transition region.
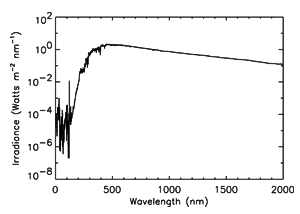
Figure 2: Solar Irradiance: 1 nm to 2000 nm